Extracellular Vesicles: Bringing Drugs from the Lab to Patients
An overview of Extracellular Vesicles (EVs), their current developmental status, and how they can be a viable delivery mechanism.
With the use of CRISPR and many other gene editing technologies, the scientific community has the impressive ability to edit cells. However, we still have a massive obstacle preventing us from realizing the full potential of these gene-editing systems. The issue is that we struggle to reintroduce these cells back into our bodies. We have all the knowledge of how a disease works but if we actually want to treat genetic diseases, we need to figure out how to replace the defective gene with a functional copy of it.
Currently, our solution lies in designing either viral or non-viral vectors that can successfully deliver the genetic material into our cells and replace these defective genes.
Viral Approach
Using viruses as a vehicle intuitively makes sense, after all, they are already good at entering our bodies and spreading. In order for viruses to be used as a vehicle, the virus is altered in a laboratory so that it can no longer infect you and cause a disease. The two types of viruses that are currently being used are adeno-associated viruses (AAVs) and lentiviruses.
Adeno-associated viruses are relatively small viruses that cause only really rare diseases. They are used as vectors because of their broad tissue tropism, which just means that they can infect a wide range of cell types including both dividing and non-dividing cells. This characteristic is extremely useful because their ability to infect a wide range of cells also means they can treat a wide range of cells if they are reprogrammed.
Lentiviruses cause chronic and deadly diseases characterized by long incubation periods, meaning that the time between when you're infected with something and when you might see symptoms is relatively long. Lentiviral vectors are thus modified to have their pathogenic components removed and replaced with therapeutic genes. Pathogenic components are just the parts of the disease that actually handle its development in our body, meaning that the disease is perfectly safe to be used after its removal. Similar to AAVs, Lentiviruses can also efficiently infect a wide range of cell types.
So what is stopping us from using these and curing all of our genetic disorders? The issue lies in the fact that our immune system still sees these viruses as threats even if their pathogenic components, meaning that they are primed to destroy them as soon as they enter our bodies. Furthermore, we are also limited in the size and type of genes that we can store inside viruses; this means that while they can enter and infect various types of cells, they may not be able to treat these cells because they cannot store the required gene.
Non-Viral Approach
The two most common approaches are Lipid and Polymer-based vehicles. Lipid vehicle, specifically synthetic lipid nanoparticles (LNPs), are the most widely used method for delivering RNA. LNPs were the vehicle of choice for both the Moderna and Pfizer mRNA vaccines. LNPs essentially form a shell around their cargo, ensuring that the gene is safely delivered to its destination. Polymer-based vehicles, on the other hand, are still a nascent technology as the only FDA-approved uses of it have been for targeted delivery of small molecules. Similar to LNPs, Polymer-based vehicles form cages around their cargo.
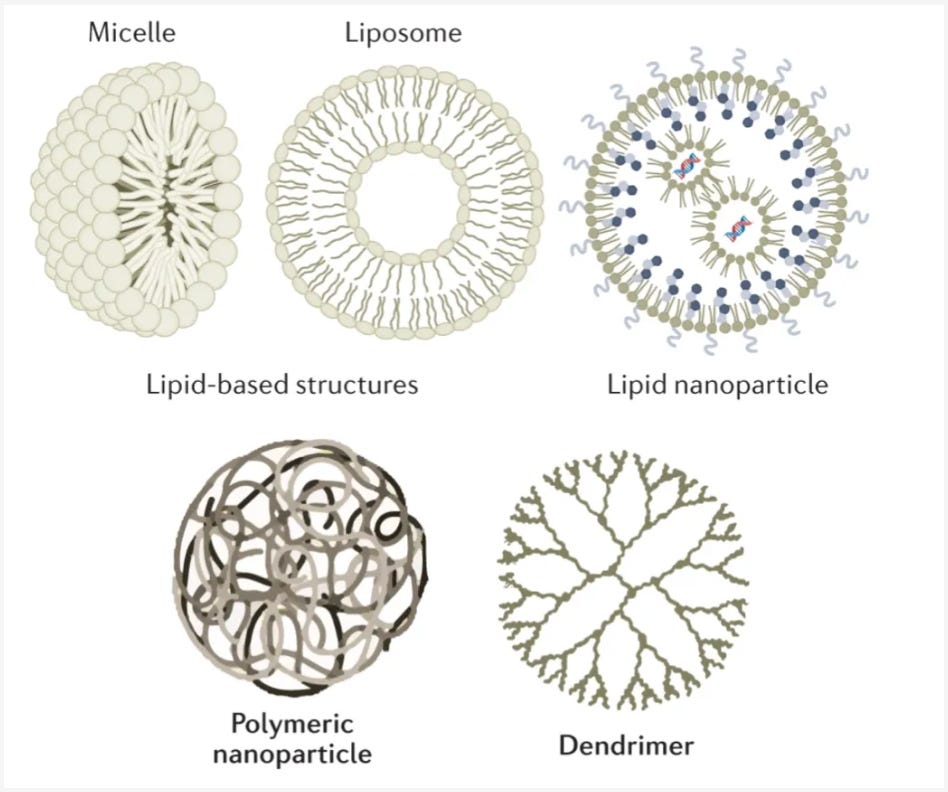
However, similar to viral approaches, these non-viral methods also have their own problems. For example, LNPs notoriously get soaked up by the liver, which has evolved to filter out these types of particles. Because of this issue, less than 5% of the particles reach the targeted disease tissue. One promising approach to address these shortcomings is to use extracellular vesicles (EVs), which can function as the cell’s own shuttle system.
EVs
EVs are small particles that are released by various cells in our body. Their primary function is as a form of intracellular communication. Extracellular vesicles can hold a wide variety of cargo, including proteins, lipids, nucleic acids (such as DNA, mRNA, and non-coding RNAs), and metabolites. These cargo molecules can be transferred to recipient cells, where they elicit specific biological responses.
EVs are classified into three main types:
Exosomes
These are the smallest vesicles, typically ranging in size from 30 to 150 nanometers
Microvesicles
These range from 100 to 1,000 nanometers
Apoptotic bodies
These are the largest, reaching sizes of several micrometers
EVs show so much promise because they already serve the core function that we want other vesicles to perform. Their role as a form of communication means that they are already programmed to enter other cells and drop off cargo. Furthermore, their numerous types also allows for some programmability, where we can pick and choose which of the EVs to use based on the size of the cargo.
One project attempting to use EVs was Codiak BioSciences.
Founded in 2015 as a merger between two separate venture creation efforts by ARCH Venture Partners and Flagship Pioneering, two of the biggest players in the biotech company creation space, Codiak had the goal of dramatically improving exosome therapeutics along three major axes:
Precision engineering
Developing systems to incorporate multiple molecular cargos into exosomes
Manufacturing
Building a scalable system that can produce exosome medicines for a large patient base
Targeting
Engineering exosomes to display surface molecules in order to enhance their delivery to specific disease tissues
Unfortunately, Codiak filed for bankruptcy in March of this year. Despite their failure, Codiak serves as an exemplar of what needs to be done before EVs can be widely adopted.
Firstly, before the EVs can be introduced into our bodies, the therapeutic proteins must be effectively loaded into the EVs, not bound or attached to their surface but instead present in a soluble form (able to be dissolved in certain fluids) inside the vesicles. They also need to be in an active and functional state, meaning that they are ready to perform their intended function.
It is also necessary to focus on the delivery of EV cargo.
While it has been demonstrated that EV cargo can arrive inside cells functionally intact (this will be discussed later), the problem is that they often end up inside cellular compartments called endosomes.
We must figure out a way for therapeutic proteins to escape from these endosomes and enter the main fluid-filled part of the cell known as the cytosol, which can later fuse with lysosomes. This results in them being chewed up and degraded.
This is crucial because it allows the therapeutic proteins to reach the cellular machinery where they can perform their therapeutic actions and produce the desired effects.
Thus, it becomes imperative to figure out how to better introduce EVs into our bodies to both improve efficacy and patient safety.
Finally, the last major area of improvement is research. As Codiak demonstrated, we do not yet have the toolset to properly study EVs and invest in products in order to make EVs work. Perhaps it would be better to begin to study a specific subclass of EVs such as exosomes, which are formed within multivesicular bodies in the endocytic-exocytic cycle and released from the cell. The endocytic-exocytic cycle just refers to the process by which cells ingest and release part of its contents. Endocytosis refers to the cellular ingestion process where cells are continually taking in small sips of their environment by pulling in small portions of their own membrane. For example macrophages eat 25% of their own volume of fluid every hour.
On the other hand, exocytosis refers to the process by which the contents of a cell are released. This process is necessary in order to balance out the endocytosis process and make sure that cells maintain a constant volume and membrane size. Essentially the whole process works where cells internally form payloads that go to the cell membrane. In the membrane, the cargo is either incorporated into the cell surface (endocytosis) or secreted into the environment (exocytosis).
Refocusing on EVs, STRM.BIO and other companies are focusing on the study of microvesicles because they come from different types of cells, meaning that they make a flexible and adjustable choice for various gene therapy uses. Whether using exosomes or microvesicles is the solution, better delivery technology and simply more research is critical for the entire field.
There has been a flurry of research in this direction but perhaps one project that I would want to highlight is from a group at the department of Laboratory Medicine in Stockholm Sweden. The problem that they were trying to address is developing efficient EVs to deliver proteins. Protein-based therapeutics would be a key development due to their unique potential to treat a variety of diseases.
Problem:
Extracellular vesicles (EVs) are promising tools to transfer very large therapeutic molecules to recipient cells. However, functional intracellular (within a cell) protein delivery by EVs remains challenging. The main issue is that the cell's outer boundary, known as the plasma membrane, naturally acts as a barrier and does not allow easy passage of proteins or other large molecules.
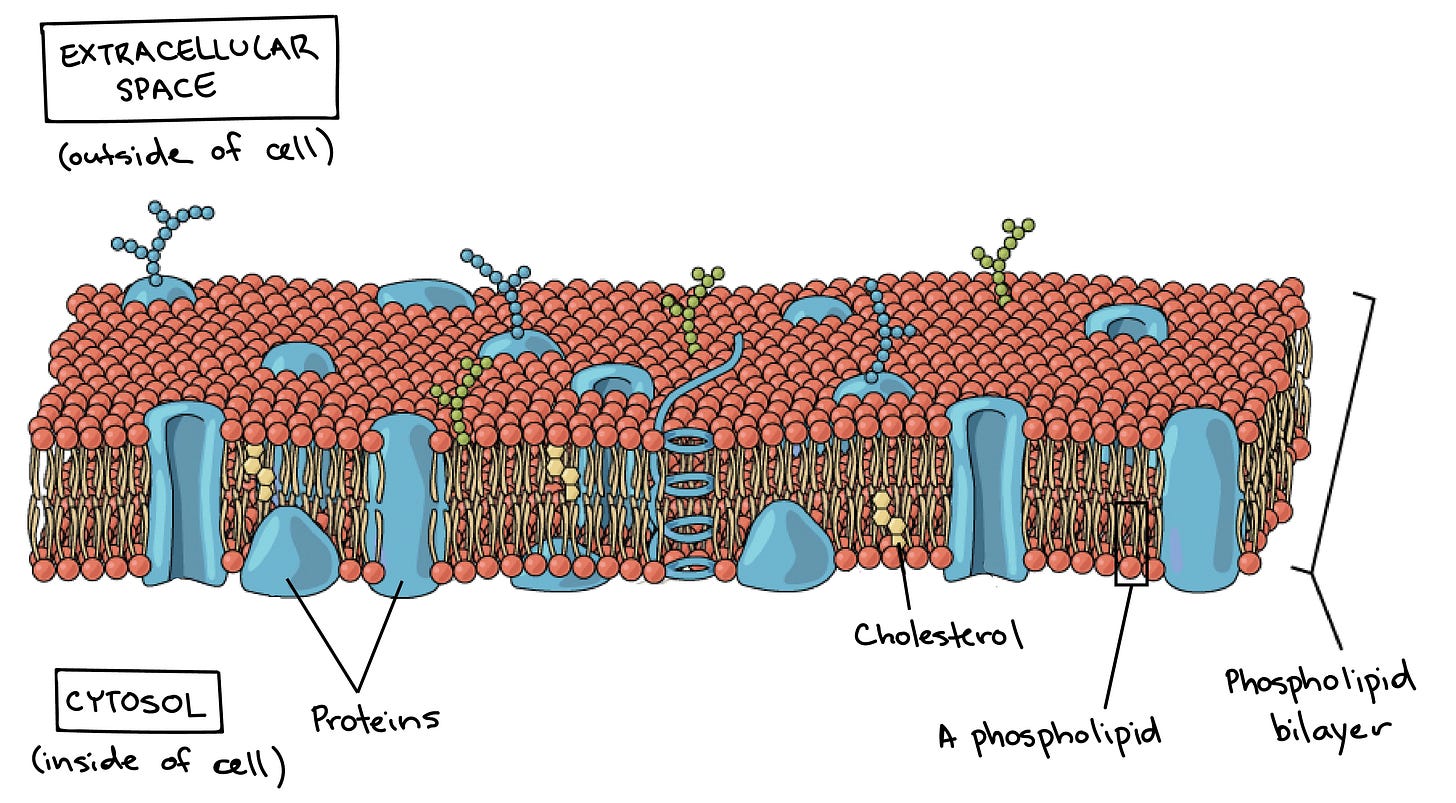
Currently, the strategies employed to solve this issue have been:
The iTOP system uses a combination of high salt concentration (NaCl-mediated hyperosmolality) and transduction compounds that help deliver proteins efficiently into primary cells. The purpose of transduction compounds is to facilitate the process of transduction or the process by which foreign genetic material is introduced into a cell. However, this method is not well-suited for use in living organisms (in vivo applications)
Another approach has been to use cell-penetrating peptides (CPPs) to deliver proteins into cells. While they have shown promise in some scenarios, they face challenges such as getting trapped in cellular compartments called endosomes and causing toxicity. If you remember, this was a similar issue with categories of EVs such as exosomes in that they would also mix with endosomes and lose their cargo.
The final mentioned approach has been the use of various nanocarriers such as lipid nanoparticles (LNPs) and polymers. They encounter similar limitations as CPPs which has also been discussed earlier.
One major drawback of all of these strategies is that their synthetic properties induce various side effects when they are applied into living organisms. This is yet another reason for why EVs are the most promising solution. Since they are a natural delivery vehicle that is already produced by our body, no side effects will occur when they are used because our body is accustomed to their presence.
Solution:
As mentioned earlier, it has been shown that EVs can successfully get through the cell membrane functionally intact. The way that the lab did this was by creating new and flexible systems that use specific tools at the molecular level to modify EVs in such a way that they can efficiently deliver proteins inside cells. This works both in a controlled environment outside of a living organism (in vitro) and inside a living organism (in vivo). They created two new systems, called VSV-G plus EV-sorting Domain-Intein-Cargo (VEDIC) and VSV-G-Foldon-Intein-Cargo (VFIC).
Both systems use a specifically engineered protein called a mini-intein (hence why you see intein in the name of these systems). This protein has self-cleaving abilities which means that it can perform the cleaving process without assistance. This is significant because it allows the protein to attach cargo to a specific region on the extracellular vesicle called the EV-sorting domain. This cargo is then later released from the outer membrane of the extracellular vesicle (EV membrane) into the interior space of the extracellular vesicle, which is called the EV lumen. They also use a protein called VSV-G in order to allow molecules to escape from a small compartment in the cell (endosome) and be released into the main part of the cell (cytosol), where they can do their job in other cells. Overall, the group demonstrated that the combination of the self-cleavage intein, fusogenic protein (VSV-G), and the EV-sorting domain are key to creating an efficient intracellular delivery mechanism for proteins.
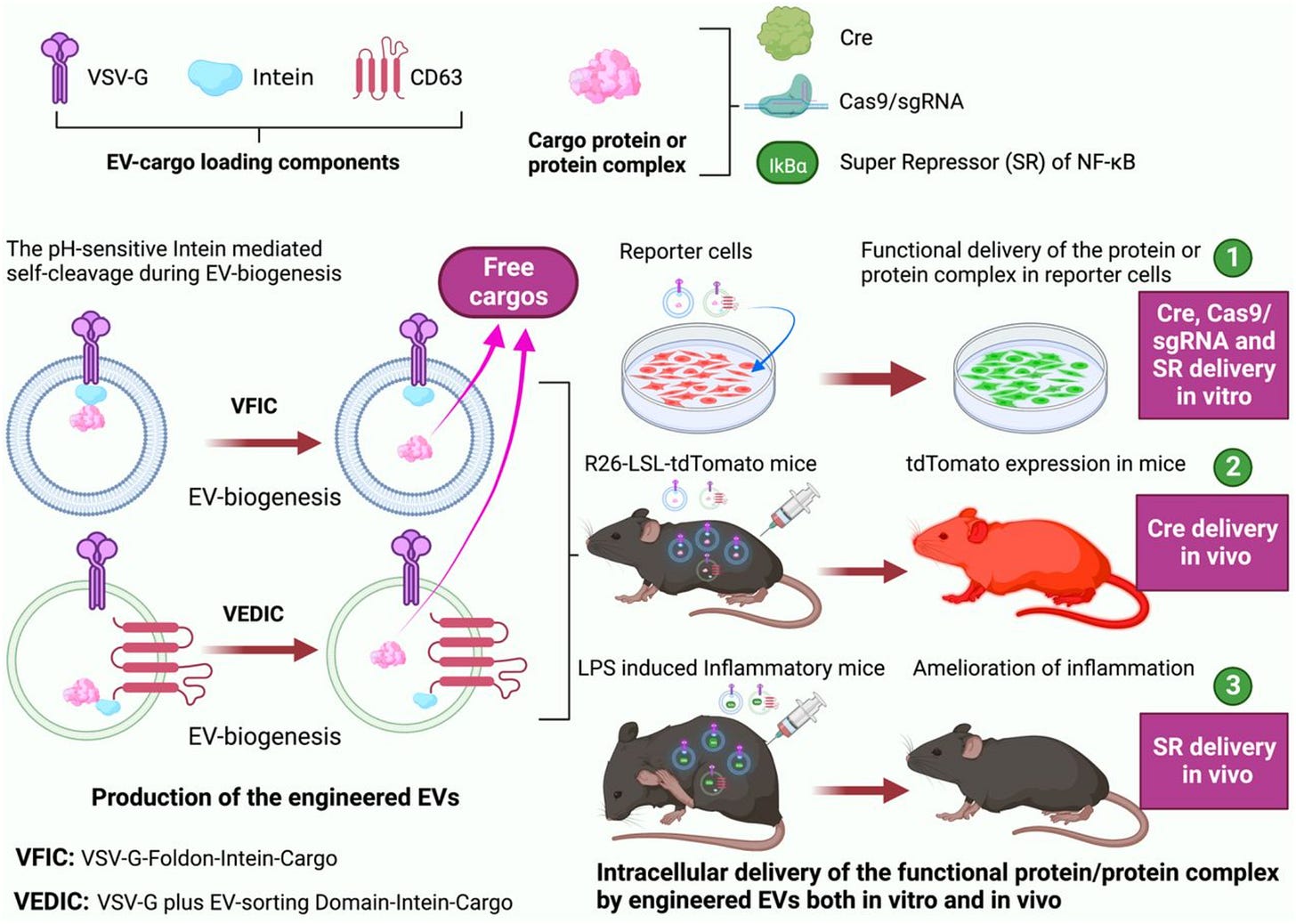
The results of their model further support this claim:
100% recombination and close to 80% genome editing efficiency in the target cells were observed by EV-transferred Cre recombinase and Cas9/sgRNA RNPs respectively. Recombination is the process of rearranging genetic material, especially by artificially joining segments of DNA from different organisms.
Specifically after injecting the modified EVs (VFIC or VEDIC), which carried a substance called Cre, directly into a specific area or throughout the body of mice, a significant change occurred inside the body of these mice.
The researches further used these engineered EVs to deliver a powerful substance that can help treat certain types of inflammation.
Altogether, the fact that the engineered EVs were able to deliver large therapeutic substances into cells demonstrates their potential to be used to treat diseases. I for one am certainly excited for a future where genetic diseases are a thing of the past and EVs are leading the way in disease treatment.
Sources:
https://www.biorxiv.org/content/10.1101/2023.04.30.535834v3.full
https://centuryofbio.com/p/cellular-shuttle-systems